The Application of Viral Vector——Liver Metabolism Research
Liver Metabolic Disease Research Model and Liver Cell Gene Transduction
Background of Liver Metabolism
The liver is the centre metabolic organ in the human body. It is involved in many physiological processes such as metabolism, bile secretion, energy conversion, detoxification, blood coagulation, and immunologic function . It is also closely related to the occurrence and development of many hereditary diseases, metabolic diseases and infectious diseases. Abnormal liver metabolism would lead to a variety of diseases, such as hepatitis, liver fibrosis, liver cirrhosis, fatty liver, liver cancer, etc. In addition, many diseases such as diabetes, lipid metabolism diseases, and hemophilia are also associated with abnormal liver metabolism. As illustrated in Figure 1, liver metabolism can be divided into the following categories:
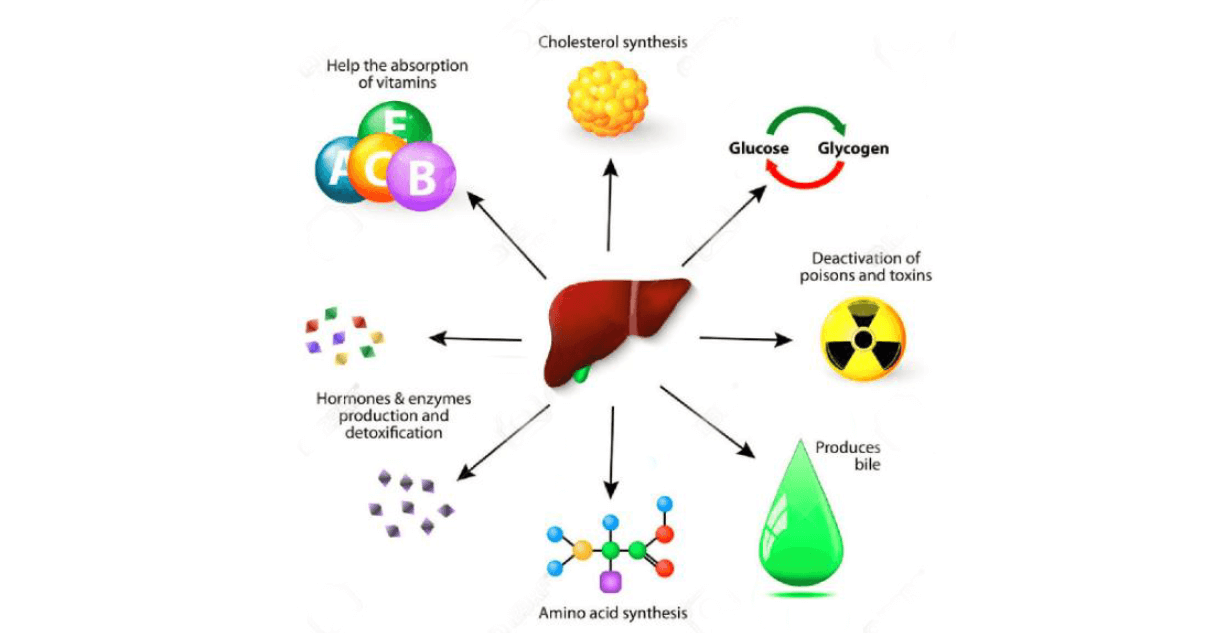
1) Liver protein metabolism synthesized proteins enter the blood circulation for the needs of systemic organ tissues. The liver produces most plasma proteins, including albumin, and produces clotting factors. The liver synthesizes ammonia from the metabolism of amino acids and excretes it through the kidneys.
2) Hepatic glucose metabolism
The liver plays an important role in regulating blood sugar levels. After a meal, carbohydrates are broken down into glucose in the small intestine, and glucose is absorbed into the blood. Glucose is transported directly to the liver after absorption. The liver removes excess glucose from the blood and stores the glucose as glycogen. Between meals, hepatocytes break down glycogen and release glucose back into the bloodstream for use by other cells of the body. If the body needs more glucose than glycogen stored in the glycogen, the liver produces glucose from other molecules such as fatty acids and amino acids.
3) Lipid metabolism
The liver plays an important role in the metabolic processes of lipid synthesis, absorption, decomposition, digestion and transportation. The liver can secrete bile, which is a conversion product of cholesterol in the liver, which can emulsify lipids and promote lipid digestion and absorption. The liver is the main site for oxidative decomposition of fatty acids, and it is also the main site for the formation of ketone bodies in the human body. The liver is also the main site for the synthesis of fatty acids and fats, and is the most abundant organ for the synthesis of cholesterol in the human body. The liver is also an important organ for the synthesis of phospholipids. The synthesis of phospholipids in the liver is closely related to the synthesis and transport of triglycerides. Impaired phospholipid synthesis leads to accumulation of triglycerides in the liver, which would contributed to developments of fatty liver.
4) Waste metabolism
The liver can process bilirubin, an insoluble decomposition product of hemoglobin. Hemoglobin is the main protein in red blood cells, is metabolized to bilirubin in the liver, then secreted in bile, and excreted into feces or urine out of the body.
5) Detoxification
The liver is the main organ for the detoxification of drugs and poisons. Hepatocytes have a variety of enzyme systems that break down foreign molecules into water-soluble compounds that can be excreted. In most cases, the liver metabolizes foreign molecules into less toxic compounds.
6) Liver and vitamin and mineral storage
The liver stores fat-soluble vitamins A, B12, D, E, and K as well as minerals, iron, copper, etc. The liver stores vitamins and minerals after digestion to meet other cells for specific biological functions.
Liver cells
Liver cells include two types: parenchymal cells and non-parenchymal cells. Hepatic parenchymal cells refer to cells with liver function, while non-parenchymal cells include hepatic stellate cells, Kupffer cells, and vascular endothelial cells. Studies have shown that hepatic parenchymal cells maintain liver homeostasis through a high-dimensional interaction network of ligand-parenchymal receptor-transcription factor-target genes secreted by non-parenchymal cells. Hepatic metabolic diseases are mainly caused by over-expression or under-expression of certain genes, and gene therapy can introduce foreign genes into diseased cells and function through transgenic expression products to achieve therapeutic purposes. Since hepatocytes are strong in protein synthesis and secretion, transduction of foreign genes into hepatocytes can regulate the synthesis and secretion of their functional products to achieve therapeutic purposes. It is an effective target cell for gene therapy and is currently clinically There are related applications, such as treatment of hemophilia and innate metabolic abnormalities. The gene transduction vector system currently applied to hepatocytes includes both a viral vector system and a non-viral vector system. Compared with viral vectors, non-viral vector-mediated gene delivery transfection efficiency is low, and sometimes leads to short-lived gene expression. As of 2015, viral vector-mediated gene transduction accounts for about 70% of global gene therapy clinical trials.
Animal Models Commonly Used in Liver Metabolism Research
Liver injury is divided into fatty liver, liver fibrosis, cirrhosis and liver cancer according to progress (Fig. 2). Fatty liver and liver fibrosis are reversible, but the damage can be irreversible once it develops into cirrhosis and liver cancer.
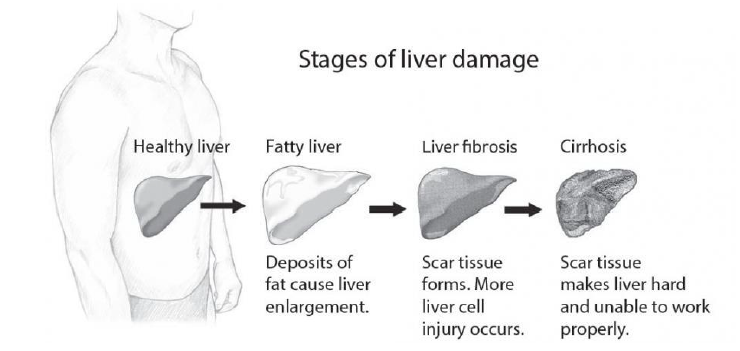
Liver damage can be divided according to the different inducers:
Chemical liver injury model
At present, most of the reagents used are carbon tetrachloride (CCl4), dimethyl nitrosamine (DMN), diethyl nitrosamine (DEN), thioacetamide (TAA), etc.
CCl4 is a typical hepatotoxic substance with a complex mechanism of action, which has been shown to be associated with oxidative stress and lipid peroxidation (Figure 3). When CCl4 enters the liver cells, it is activated by cytochrome P450 enzymes to produce trichloromethyl free radicals(CCl3·)and trichloromethyl free radicals(OOCCl3·). These free radicals are associated with hepatocyte membrane and endoplasmic reticulum, Covalently binds to phospholipid molecules on mitochondria, triggering lipid peroxidation to damage the structure and function of the membrane, and inhibiting the activity of calcium pump on the cell membrane and mitochondrial membrane, causing a large amount of Ca2+ influx, leading to liver cell damage, thereby affecting Normal cell energy metabolism. By-products of lipid peroxidation, such as reactive aldehydes, can also bind to normal intracellular proteins and DNA, and have hepatotoxicity and carcinogenesis. In addition, CCl4 metabolites can also stimulate liver Kupffer cells to release pro-inflammatory cytokines, further aggravating liver damage. After the structure and function of the liver cell membrane are damaged, intracellular transaminase ALT and AST overflow, which increases plasma ALT and AST. This reflects the degree of damage of hepatocytes to some extent. Therefore, elevated plasma ALT and AST are considered to be judged. An important indicator of the severity of acute liver injury. The model of liver fibrosis induced by CCl4 is characterized by simplicity, low cost, short modeling period and high similarity to human liver fibrosis.
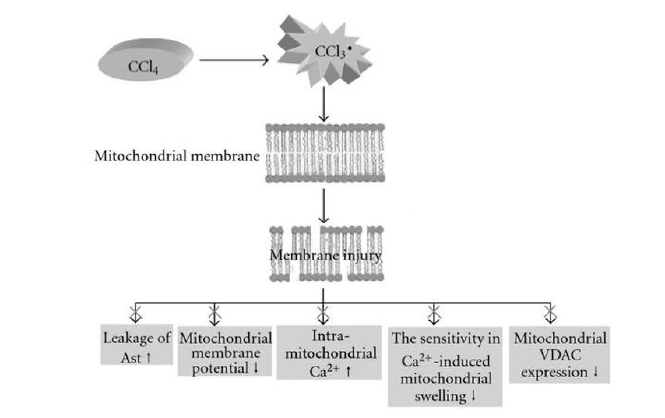
DMN is a common hepatocarcinogen. It is metabolized by microsomes. The combination of intermediate products with cellular nucleic acids and proteins can cause intracellular macromolecular damage and induce the activation of hepatic stellate cells in the hepatic sinus wall, resulting in excessive increase and deposition. It causes the formation of liver fibrosis, and promotes the formation of sinusoidal capillary sinus capillaries, aggravates liver damage and promotes the development of liver fibrosis. Intravenous injection of 15ul/kg DMN resulted in acute liver injury, which resulted in prolonged hepatic prothrombotic enzyme activity, a significant increase in alanine aminotransferase, and decreased glutathione content and superoxide dismutase activity. Intraperitoneal injection of 1.5% DMN1ml/kg, 3 times/week for 4 weeks can cause extensive necrosis, fibrous tissue hyperplasia, liver atrophy, decreased serum protein concentration, and elevated total bilirubin in rat hepatocytes. And cirrhosis. DMN is an alkylating agent. Hepatocyte experiments in vitro showed that DNA damage caused by DMN treatment for 6h resulted in cell death after 24h. DMN also caused endothelial cell damage and altered morphology of adjacent hepatocytes.
DEN is a strong carcinogen that binds to DNA and RNA and causes liver damage. Commonly used 0.01% DEN solution, free to drink water, change once a day, continuous drinking for 11 weeks can form cirrhosis. Studies have given rats 0.01% DEN solution (1-5 weeks for DEN solution, 6-8 weeks for DEN-free water, 9-20 weeks for DEN solution), 14th week for cirrhosis model, weeks 15-20 Cancerous cells were found. In addition, Wistar rats weighing approximately 250g were given an intraperitoneal injection of 100 mg/kg DEN solution once a week to form cirrhosis at the 8th week. The method not only shortens the molding time, but also has a molding rate of 100%, but its toxicity needs to be protected in actual operation.
TAA has a direct hepatotoxic effect. After ingestion, it can be metabolized by cytochrome p450 mixed functional oxidase in hepatocytes to TAA sulfur oxides, interfering with RNA transfer in the nucleus, affecting protein synthesis and enzyme activity, and increasing DNA synthesis and mitosis in hepatocytes. It promotes the development of cirrhosis, activates hepatocyte phospholipase A2, destroys the liver cell membrane, and forms intestinal endotoxemia, which leads to the destruction of large areas of liver cells and can significantly increase ALT and AST. Small doses of TAA induce hepatocyte apoptosis, and large doses lead to lipid oxidation and central necrosis of the lobules. The degree of damage is positively correlated with TNF-γ and endotoxin levels, and can be alleviated by hydroxyl radical scavengers. TAA-induced cirrhosis was performed by intraperitoneal injection of 4% TAA solution at 0.2 g/kg·d, 3 times/day for 10 weeks, and hydroxyproline was significantly elevated in liver tissue, collagen and transformation. The mRNA expression of growth factor β1 was significantly increased, hepatocyte necrosis, regenerative nodule formation, capillary bile duct hyperplasia, portal hypertension and cirrhosis.
Immunological liver injury model:
Rats are usually injected intraperitoneally with human serum albumin and pig serum. This modeling method can well replicate the possible processes and mechanisms of cirrhosis caused by human autoimmune liver disease, and is commonly used in the screening and evaluation of anti-cirrhosis immunity drugs. At present, pig serum is the most commonly used immune antibody. Rats weighing 150-190 g are given intraperitoneal injection of 0.5 ml of pig serum twice a week for 7 weeks. After 7 weeks, a large number of fibroblasts proliferated and pseudolobules appeared. The modeling method is characterized by the fact that it is similar to the formation of human liver fibrosis, and the hepatocytes are not damaged during the fibrosis process, and the fibrosis is longer than the liver fibrosis caused by the CCl4 method. It is suitable for thestudy of the mechanism of liver fibrosis production and the screening of immunomodulatory drugs. However, the modeling time is long, and the animal mortality caused by allergic reactions is high.
Alcoholic liver injury model:
The liver is the main site of alcohol metabolism, and the alcohol that enters the liver is converted to acetaldehyde. Acetaldehyde and its transformant acetic acid damage the structure and function of the liver through a variety of pathways leading to a sustained inflammatory response. At the same time, the liver's fat oxidation is weakened, fatty liver is formed for a long time, and finally liver fibrosis is formed. At present, the commonly used model preparation methods are gavage method and rat free drinking alcohol-containing aqueous solution. The method of intragastric administration was administered with a mixture of “white wine-pyrazole-vegetable oil”. Each rat was white wine 48g/(kg*d), pyrazole 24g/(kg*d), corn oil 2ml/(kg*d). The mixture was administered with a slight alcoholic liver fibrosis after 16 weeks. It is difficult for rats to have alcohol and it is difficult to drink freely. The white wine is diluted into (6%, 9%, 12%, 20%) and other aqueous solutions of different concentrations, and as the only water source of the rat, the 6% white wine aqueous solution is firstly used to gradually adapt the rat to the smell of white wine, and then according to Gradual increase and decrease of concentration after adaptation, liver fibrosis appeared after 16 weeks. The models prepared by the two methods are similar to human alcoholic liver fibrosis. In comparison, the method of free drinking white liquor is simpler, the mortality is lower, and the cost is lower, but the gavage method ensures each large The alcohol intake of the rats has high modeling stability. Ethanol can increase the liver oxygen digestibility of animals, increase the oxygen consumption of sinusoidal blood, thereby reducing the oxygen supply to the hepatocytes of the lobules, causing the degeneration and necrosis of hepatocytes in this area, while necrosis and inflammation are the formation of collagen fibers. Factor, the currently commonly used method of administration is to administer a certain concentration of ethanol while feeding the solid feed.
High fat diet induced non-alcoholic fatty liver injury model:
The incidence of nonalcoholic steatohepatitis (NASH) is gradually increasing in China, and the mechanism of liver fibrosis is: excessive fatty acid in the liver, which is deposited in the liver, leading to hepatic steatosis. Moreover, fatty acids can activate hepatic stellate cells through Kupffer cells and participate in the formation of liver fibrosis. At the same time, elevated cholesterol in the blood produces more inflammatory factors, which cause inflammation and fibrosis. The modeling method is to take a rat with a body weight of 120-160 g, and provide sufficient high-fat feed (82.5% ordinary feed, 2% cholesterol, 10% lard, 5% egg yolk powder, 0.5% sodium cholate) daily. Free drinking water. The model for the diagnosis of moderate fatty liver was formed at 8 weeks of modeling, and the appearance of liver fibrosis was observed. After 12 weeks, the pseudolobules were obvious and liver fibrosis was formed. This modeling method is similar to the natural occurrence of NASH fibrosis in humans, and it is short-lived, easy to operate, low mortality, and low cost of modeling. It is a better animal model for clinical research of NASH liver fibrosis.
Bile duct occlusion liver fibrosis (BCM) model:
The BCM model is prepared by artificially causing extrahepatic biliary obstruction by cutting the bile duct (Fig. 4) or injecting a sclerosing agent, thereby causing bile duct dilatation and cholestasis above the obstruction site, and increasing pressure in the biliary tract, causing extravasation of bile, resulting in liver Cell lysis and necrosis. In addition, because the intrahepatic blood vessels are compressed by the dilated bile duct, it can also cause ischemia and necrosis of hepatocytes, and the collagen tissue is deposited in a large amount, extending into the hepatic lobules, surrounding the hepatic lobules and spreading around the hepatocytes, thereby forming liver fibrosis. Biliary duct obstruction was induced by bile duct ligation and severing and retrograde injection of N-butyl-2-cyanoacrylate bile duct in rats, and liver fibrosis occurred at 28 days. The BDL-induced liver fibrosis model has a short molding time and low cost, and its pathogenic factors are very similar to clinical cholestatic liver fibrosis (Fig. 5). Therefore, the model is used to prevent liver fibrosis, especially bile. It has important value in the evaluation of the efficacy of silicosis liver fibrosis. However, the mortality rate of this model is high and the operating environment is high. Moreover, since the model damages liver tissue by causing cholestasis by ligating the common bile duct, the model is not suitable for evaluation of drug effects exerted by choleretic effects.
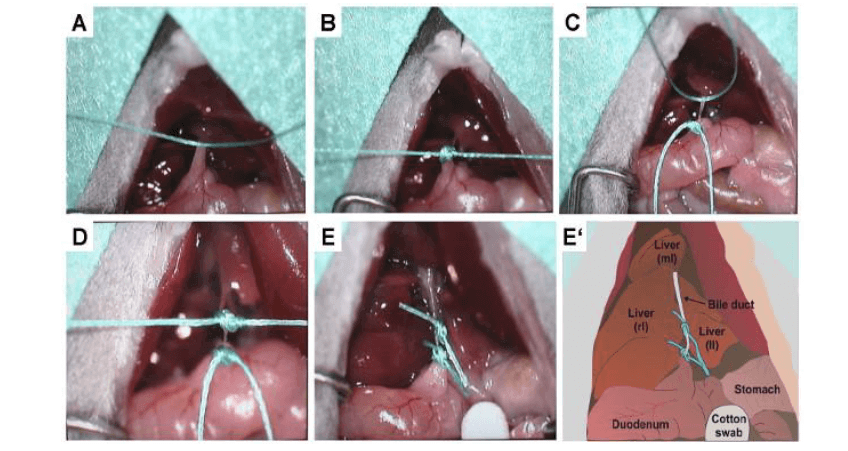
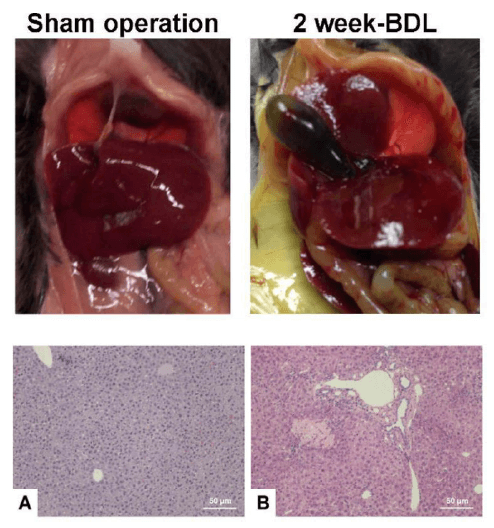
The Application of Viral Vectors in Liver Gene Transduction
Viral vector
The current four major viral vectors are: adenovirus, lentivirus, retrovirus, adeno-associated virus (AAV). Among them, retroviruses are basically only used for blood/immune system research.
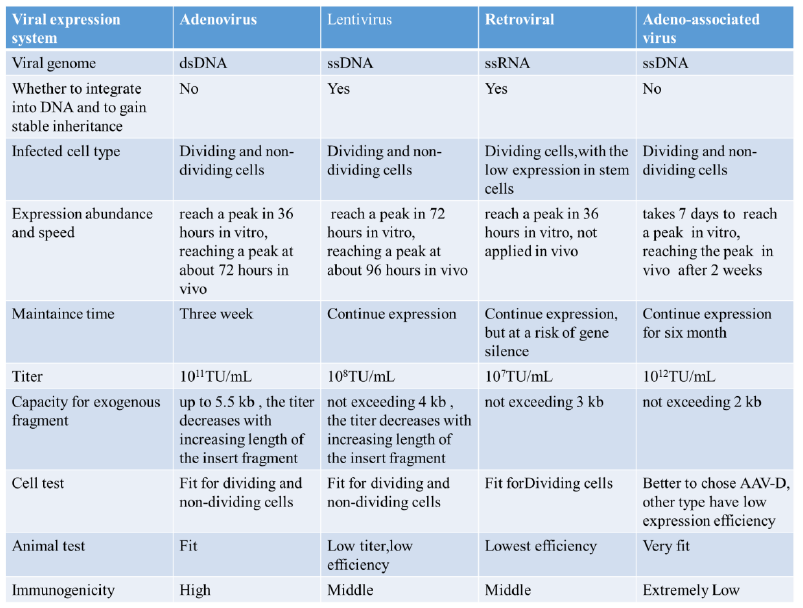
The table below compares the four virus systems. In the study of liver metabolism, lentiviruses and retroviruses are rarely used, and they are used in the transduction of some in vitro cell lines, while glands are commonly used in primary hepatocytes, primary hepatic stellate cells, and in vivo hepatocytes. Virus and AAV. In the early articles, liver gene transduction was mostly mediated by adenovirus, but because adenovirus produced a strong immune response, it easily caused liver damage, and had a non-negligible effect on liver metabolism, so the use of adenovirus was increasingly affected. Limitations, AAV-mediated liver gene transduction, which has been developed in recent years, has lower immunogenicity and longer expression time, so more and more studies tend to use AAV vectors. AAV has multiple serotypes. The main difference between AAV vectors of different serotypes is that the capsid proteins are different, so there are differences in transfection efficiency for different cells and tissues.
The main subjects of liver metabolism research include hepatocyte cell line, hepatic stellate cell line, primary hepatocyte, primary hepatic stellate cell, and in vivo liver research. The scores of different viral vectors in liver transduction in vitro and in vivo are shown in Table 1.
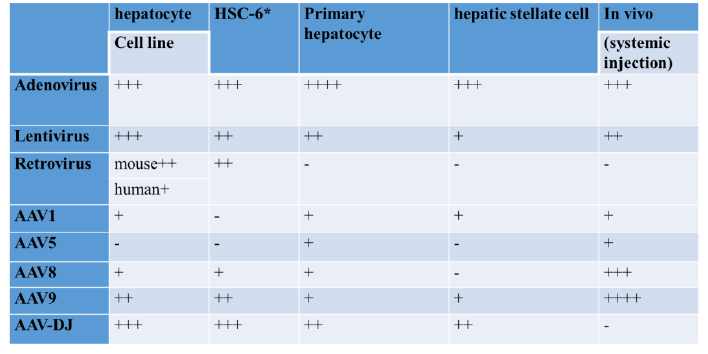
The advantages and disadvantages of comparing adenovirus, lentivirus, retrovirus and AAV-mediated liver gene transduction are as follows:
Adenovirus: The largest capacity of the vector, up to 6kb, can be observed after 3 days of infection; but the expression efficiency is low in the animal, the immunogenicity is high, and the immune response is easy to be induced; the larger molecule is recognized by the defense system in the body and cleared. Therefore, the expression cycle is only 2-3 weeks. Generally, the gene of interest is relatively large, and the test period is short, and liver gene transduction in the adenovirus-mediated conductor can be selected.
Lentivirus: low virus titer, low efficiency, integrated into the genome, change the host genome structure, cause the activation of proto-oncogenes and lead to adverse reactions such as liver cancer.
retrovirus: low virus titer, can only infect dividing cells, integrated into the genome, change the host genome structure, causing the activation of proto-oncogenes leading to adverse reactions such as liver cancer.
AAV: The virus particles are small in diameter, high in titer, and evenly spread in the liver; not easily detected by the immune system, low in immunogenicity, high in safety, and no pathogenicity is currently found, according to the National Institutes of Health (NIH) For the relevant rating of biotechnology products, AAV belongs to the safest grade of RG1, while adenovirus and lentiviral vectors belong to RG2, retroviral vector belongs to RG3; it can continue to express in vivo for more than 6 months, and has long-lasting effect; AAV-8 can specifically infect the liver, AAV-9 has a good effect on liver infection; it can be injected directly; mainstream magazines agree that it can improve the score of the article. The disadvantage is that the fragment size is relatively small and can currently accommodate up to 4.7 kb segments.
In Vitro Liver Gene Transduction and Viral Vector
Adenovirus, lentivirus and AAV can be used for transduction of liver gene transduction by viral vector. The results of infection of primary hepatocytes with adenovirus, lentivirus, and AAV-9, respectively, are shown in Figure 7. It can be seen that adenovirus has the highest infection efficiency, followed by lentivirus, and AAV-9 infection efficiency is relatively low. Therefore, in terms of infection efficiency, adenoviral vectors are preferred for in vitro hepatocyte gene transduction. AAV-DJ can broadly infect a variety of cells, with high infection efficiency, and can also be used for in vitro hepatocyte gene transduction.
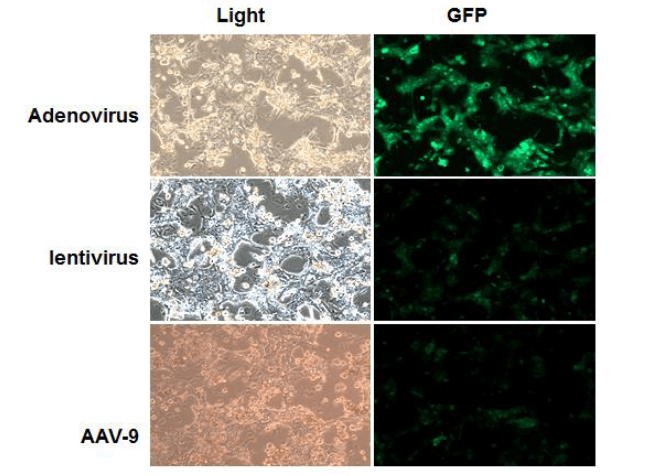
See the appendix for the method of isolating mouse liver cells in vitro.
Different AAV serotypes have different affinities for different cells. For AAV, different types of cell infection experiments first need to determine the appropriate AAV serotype, and then based on the optimal MOI.
Exploring the optimal serotype
Figure 8 shows the fraction of AAV-2-impregnated cells as a standard infection score (100 points), giving different AAV serotypes the ability to infect different cells, such as Huh-7, DJ type is 500, meaning DJ The infectivity of Huh-7 is 5 times that of AAV-2. The serotype of some cells can be determined according to the following table. However, we recommend pre-experiment to explore the optimal serotype before conducting the formal experiment.
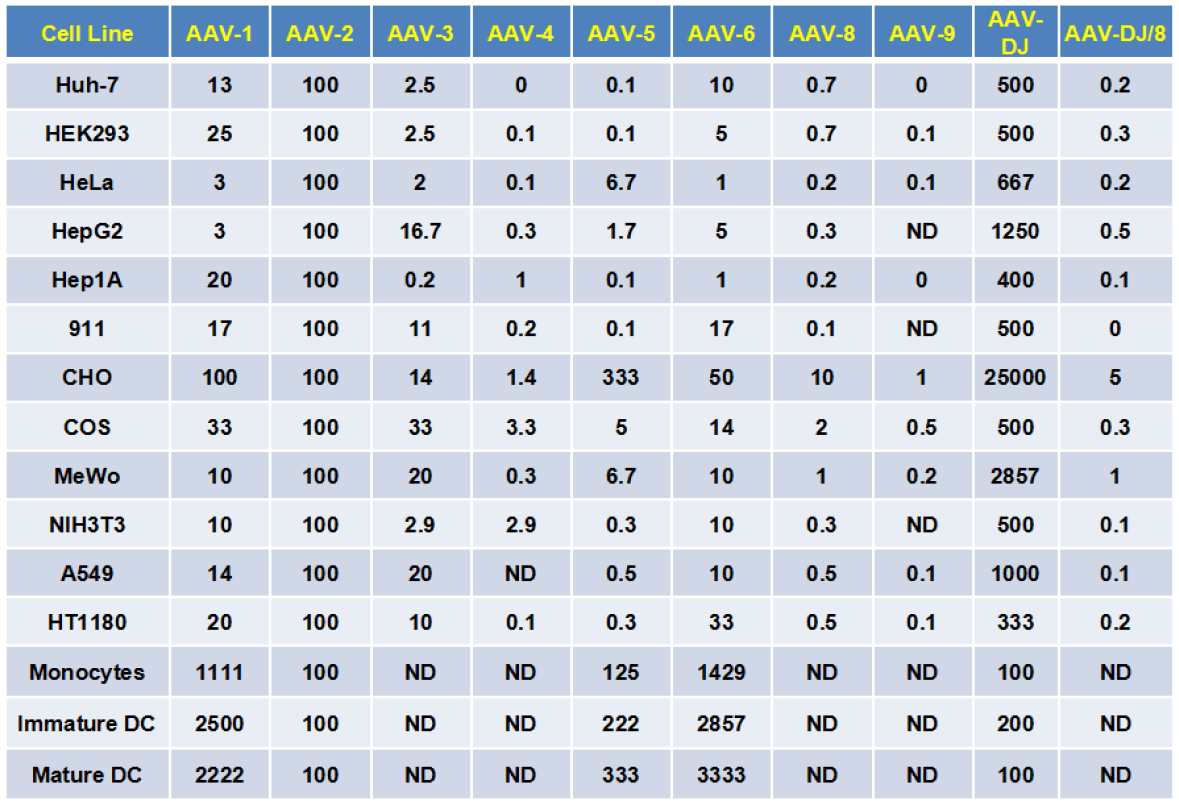
Explore the optimal MOI
MOI (Multiplicity of Infection) refers to the number of viruses infected per cell. Generally, the higher the MOI, the higher the number of adenoviral genomes retained in the host cells after AAV virus infection and the expression level of the protein of interest. AAV has a limited ability to infect isolated cells, and the required viral MOI is greater than 104. A certain infection effect can still be achieved by selecting the appropriate serotype and infecting the MOI with a suitable virus. The AAV virus has different MOIs for different kinds of cells from different sources. In principle, the optimal MOI is the lowest MOI with better infection efficiency. For each type of virus, each cell should be subjected to an optimal MOI exploration experiment. The MOI gradient exploration experiment should be set up and the appropriate MOI should be selected for the experiment. The MOI of AAV cell infection is generally explored at a gradient of 104:105:106.
The steps of AAV infecting hepatocytes in vitro are as follows:
1. Cell culture: Inoculate a good target cell into a cell culture dish, and use a small-pore culture dish (24-well plate or 48-well plate) as much as possible. The number of cells inoculated is slightly different due to the growth rate of the cells, which is generally guaranteed. The cell confluence rate was between 50% and 70% when the virus was infected the next day.
2. Conversion of MOI to virus volume The number of cells at the time of transfection (generally calculated by the number of cells counted by passage × 2) × MOI = number of viruses; the number of virus added = number of viruses / virus titer. For example, if the number of transfected cells is 105 and the MOI is 105, the number of viruses required is 1010. If the titer of the virus is 1012 v.g/ml, the volume of the virus to be added is 1010/(1012 v.g/ml). ) = 10-2ml = 10ul.
3. AAV infection of the target cells The infection experiment was divided into two groups, respectively, adding the corresponding purpose of AAV and the same titer of the same volume of control AAV, 1/2 volume of culture medium infection. The range of virus added is recommended to be MOI = 104; 105; 106. After 1/2 volume infection for 2 hours, do not change the solution, add 1/2 fresh culture solution, continue to infect 37 degrees overnight. Infected fresh liquid for 12-24 hours of infection.
4. Observing the infection After 72-96 hours of infection, GFP/RFP expression can be observed (only for AAV with GFP/RFP independent expression cassette). The infection time of overexpression and interference is best for 96 hours or more. There are also cells that need to be extended to about 1-2 weeks to observe.
In vivo liver gene transduction and viral vectors
Different from the viral gene-mediated transduction of liver gene transduction, liver gene transduction in the carrier of viral vector needs to consider factors such as safety, pathogenicity, homogeneity, specificity and expression duration. By comparing the advantages and disadvantages of liver gene transduction in different viral vector-mediated conductors (Fig. 9), AAV is the safest, uniform, long-acting, specific and efficient viral vector in liver gene transduction studies in vivo, and has unique advantages.
Comparison of injection methods: comparison of tail vein injection and portal vein injection as follows, portal vein injection requires open surgery, the operation is cumbersome and postoperative bleeding is likely to affect subsequent research. The tail vein injection is an intravenous injection of the virus from the tail of the animal, which relies on blood circulation to reach the liver. The operation is simple and convenient, and it is the most widely used injection method. Therefore, tail vein injection is well suited for AAV-mediated liver-specific gene transduction.
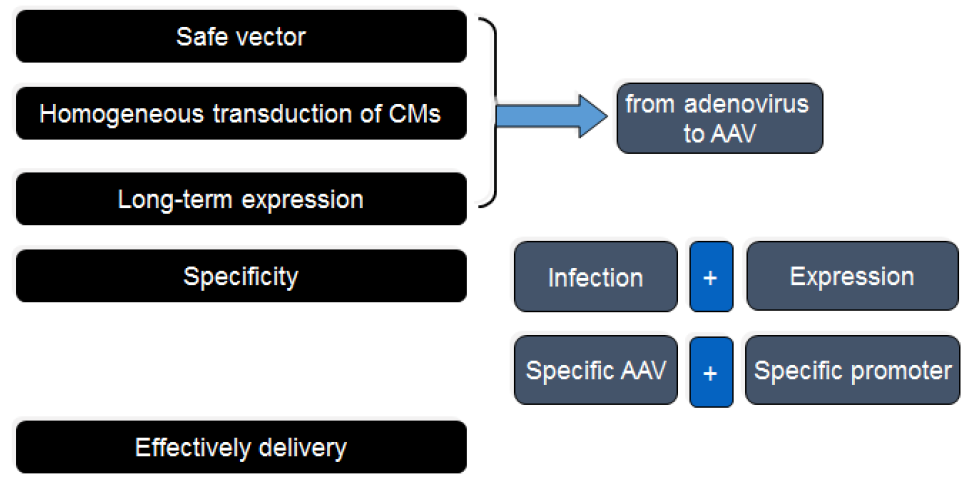
AAV-8 can efficiently and specifically infect the liver in different serotypes of AAV. AAV-9 is slightly less specific than AAV-8 infecting the liver, but its infection effect is stronger than AAV-8. Therefore, AAV-9 is used, and the combination is preferred. Liver-specific promoters and other molecular components enable efficient and specific gene transduction of the liver, providing a good guarantee for subsequent experiments.
A schematic diagram of infection of mouse liver by tail vein injection and fluorescence of liver sections of infected mice using AAV-9 (Fig. 10) showed high infection efficiency.
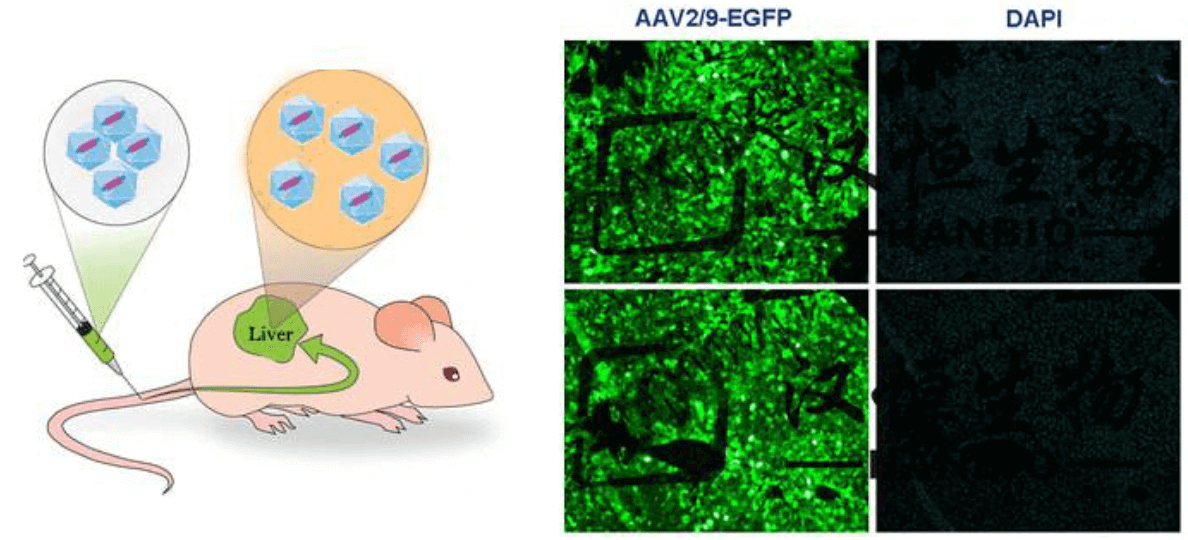
Case sharing
Case 1: The use of the AAV gene transduction vector driven by the GFAP hepatic stellate cell-specific promoter has achieved specific infection of hepatic stellate cells invivo, and the related research results have been published in the internationally renowned journal Nature Communications.
AAV-mediated knockdown and overexpression of CUGBP1 in vivo to achieve specific knockdown or overexpression of CUGBP1 in liver cells, and found that CUGBP1 is involved in the regulation of hepatic stellate cell activation and liver fibrosis. In this article, the authors constructed the AAV viral vector system to overexpress the CUPBP1 driven by the liver-specific promoter GFAP through the mouse tail vein into the BDL-induced liver fibrosis model mice, and to detect the indicators of liver fibrosis in mice ( Figure 11).
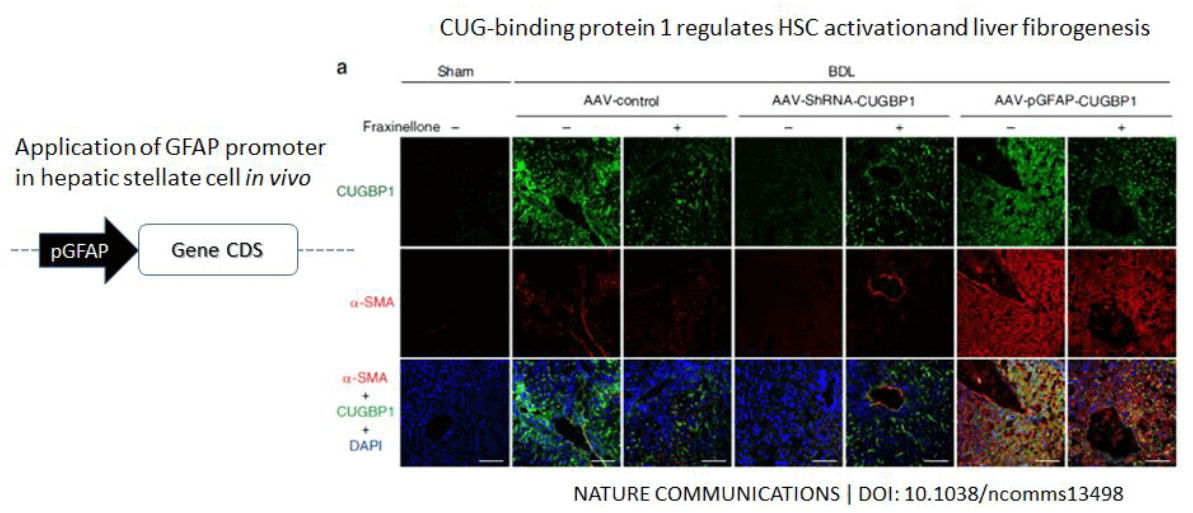
Case 2: Different fragments of some liver-specific promoters were screened with different lengths and lengths using fluorescein reporter technology. 100 μl of tail vein was injected, infection was 3 weeks, and small animals were imaged in vivo. Screening out a liver-specific promoter using TBG (Human thyroxine-binding globulin promoter, TBG promoter, abbreviated as TBG) can achieve specific infection of liver cells in mice with AAV, without non-specific organ infection (Fig. 12).
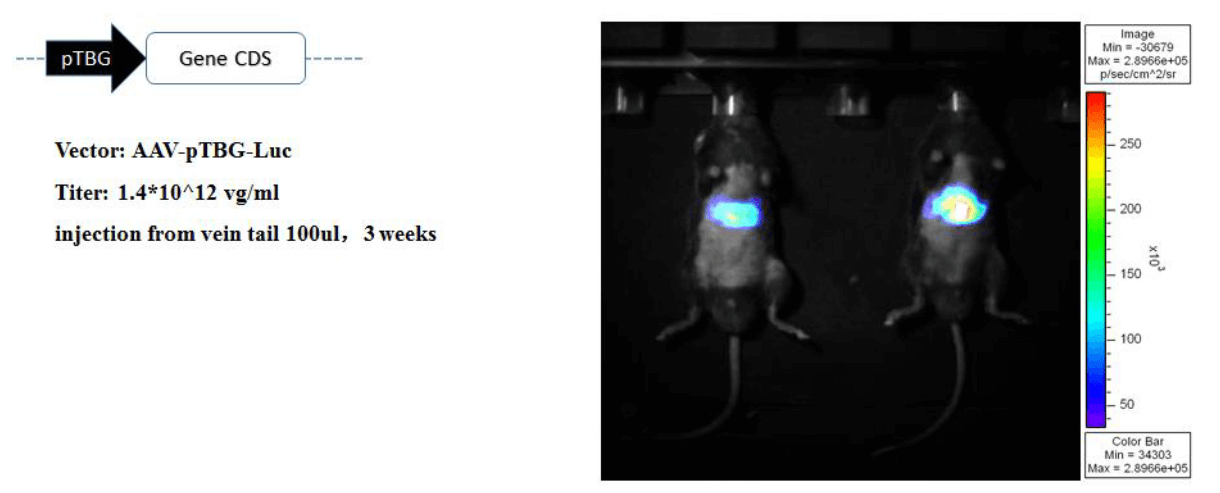
In an article published in the internationally renowned journal Cell in 2016, the overexpression of IKKβ in liver cells in mice was achieved by injection into the tail vein of mice in a high-fat-induced obesity model. Specific infection of hepatocytes in mice with AAV was achieved using the TBG liver-specific promoter in this study. AAV-mediated overexpression of IKKβ In vivo experiments have achieved specific overexpression of IKKβ in liver cells, and it has been found that IKKβ is involved in obesity-induced inflammation and can improve glucose homeostasis. In this article, the authors constructed the AAV viral vector system to transfect the liver-specific promoter TBG-driven IKKβ overexpression into the obese-induced inflammatory model mice through the tail vein of mice, and to measure the metabolic index of the mouse liver (Fig. 13).
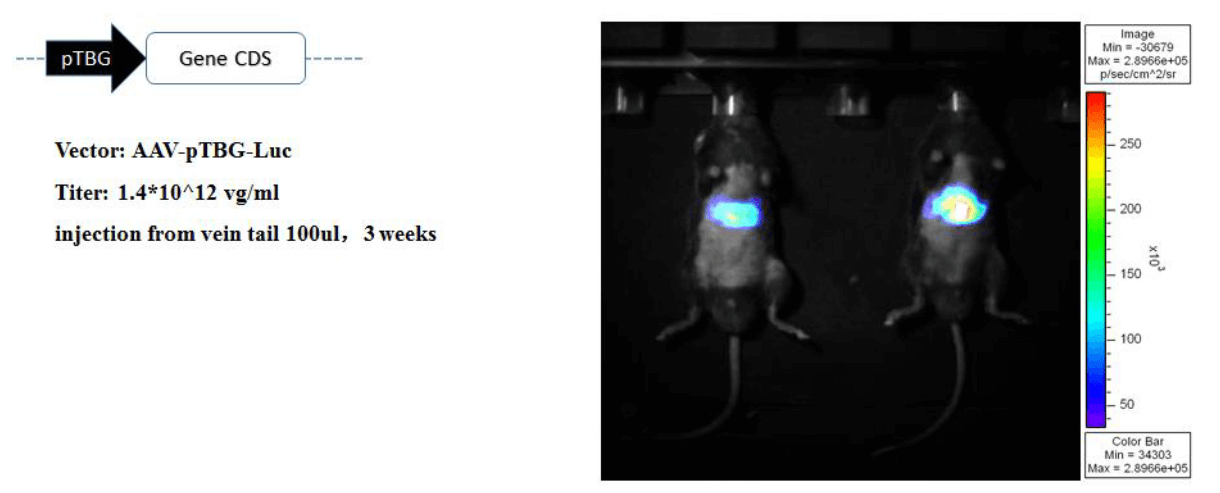