Landscape of in vitro cell transfection and in vivo transfection
(siRNA transfection,DNA/plasmid transfection, nanoparticle transfecton, electroporation, transfection protocol and so on)

Introduction of transfection
In general, transfection is the process of delivering nucleic acids, such as DNA and RNA into eukaryotic cells, resulting in the expression or production of proteins or down-regulation of the targeted protein. Although not very common, protein transfection is also used to promote the rapid expression of target protein (such as Cas9 protein transfection for CRISPR for genome editing) [1,2]. To date, transfection has been applied in the field of basic research (gene overexpression or knockdown) and translational research (such as gene therapy [3], immunotherapy and induced pluripotent stem cell generation [4]).Various delivery methods have been reported and each method has its specific properties. It’s very important to select the most effective transfection method according to the cell type, transfection efficiency, target cell viability and expression levels. Typically, the methods can be divided into two categories: non-viral and viral [5]. Non-viral transfection methods contain physical treatment (electroporation, nanoparticles, etc.), chemical materials mediated transfection (such as liposome, polymer). To deliver exogenous genes with viral methods, gene of interest should be packaged into a replication-deficient viral particle, and then the viral particles inject its DNA (including gene of interest) into target cells. To date, several types of virus have been applied for gene delivery, such as lentivirus, adenovirus (Ad), adeno-associated virus (AAV), retrovirus (Retro) and herpes simplex virus (HSV) and so on.
Content of Transfection in vitro / in vivo
- 1. Introduction of transfection
- 2. Transfection in vitro
- 3. Transfection in vivo
- 4. Small interfering RNA (siRNA) transfection
- 5. Reference
Transfection in vitro
Transfection in vitro can be mediated by the non-viral reagents (such as polymer, liposome, nano-particle) or viral vectors (such as lentivirus, adenovirus and AAV).Polymer-based transfection
Polymer transfection is a technique used to deliver DNA or RNA into cells with biodegradable cationic polymer [6], such as DEAE-dextran or polyethylenimine (PEI). The negatively charged DNA binds to the polycation and the complex is taken up by the cell via endocytosis. With some toxicity, polymer conjugated complexes can mediate transfection efficiently in some cell types, such as 293T cells, but are not suitable for transfection of sensitive cells and generation of stable cell lines.Liposome-based transfection
Liposome transfection, also known as lipofection or lipid transfection, is a technology used to deliver DNA or RNA into cells mediated by liposomes, which are tiny vesicular structures with the same composition as the cell membrane and can easily fuse with the cell membrane [7]. To date, there have been several generations of liposome, differing in the addition of cationic lipid and DNA-compacting substances. Now the most commonly used liposome is highly potent cationic lipofection reagent that has been shown to effectively transfect plasmids or siRNA, as well as nucleic acid-protein complexes, into cultured adherent and suspension cell lines [8]. Besides, the efficiency of lipofection can be improved by treating transfected cells with a mild heat shock [9]. Genemedi has launched a transfection reagent of Lipogene, which is comparable to Lipofectamine 3000, much more effective than polyethylenimine (PEI).You could find more information on this website: www.genemedi.net/i/lipogene-transfection-reagent.
Nanoparticle-mediated transfection
Non-viral gene delivery system – multifunctional envelope-type nano-devices (MEND) – has been proposed to package DNA or RNA into a nano-sized structure with programmed assembly of functional devices, enabling DNA protection from DNase, size control and improved packaging efficiency. Then polycations and lipids were added based on electrostatic interactions to realize lipid-film hydration [10]. Now Impalefection has been developed to impale cells to deliver DNA or RNA using nanomaterials, such as carbon nanofibers, nanotubes, nanorods, silicon nanowires [11]. Nano-particle transfection transfers the DNA or RNA via membrane fusion into the cell, avoiding DNA/RNA degradation by lysosome and showing great advantages over polymer and liposome.Electroporation-mediated transfection
Electroporation, also known as gene electrotransfer, is a popular method to realize high efficient transfection. During electroporation, cells and DNA/RNA of interest are exposed to short pulses of an intense electric field, leading to a transient increase in cell membrane permeability and the entry of DNA/RNA around into cytoplasm. Once removing the electric field, the cell membrane stabilizes and closes, allowing the expression of the enclosed DNA/RNA. Though electroporation-mediated transfection is easily operated and reliable, but it leads to high rates of cell death and requires great numbers of cells. Besides, a special and matched electroporation device is needed for electroporation [12].Comparison | Polymer | Liposome | Aano-particle | Electroporation |
Principle | Endocytosis | Endocytosis | Membrane fusion/impale cells | Transient increase in the permeability of cell membrane |
Integration | No | No | No | No |
Time to peak expression | 48h-72h | 48h-72h | 24h-48h | 48h-72h |
Sustainable time | Transient expression | Transient expression | Transient expression | Transient expression |
Cell Type | A number of cell types | Adherent and suspension cell lines | Almost all the cells | Not suitable for sensitive cell types |
Particle diametre | 75 to 520 nm | 50-200nm | 100-300nm | - |
Animal experiment | Low efficiency | Low efficiency | Target delivery | Target delivery |
Cytotoxicity | High | Low | Non-toxic | - |
Immune Response | No | No | No | No |
Efficiency | Low efficiency (<10%) | Depend on cell type | High efficiency | High efficiency |
Price | Inexpensive | Medium | Expensive | Most expensive (electroporation device) |
Lentivirus-based gene transduction in vitro
Lentiviral vectors can mediate efficient transfection and long-term expression of exogenous genes in both dividing and non-dividing cells and have been widely used for gene overexpression, RNA interference, microRNA research and in vivo animal experiments.More useful information about lentivirus can be found on this website: https://www.genemedi.net/i/lentivirus-packaging .
Adenovirus-based gene transduction in vitro
Based on human adenovirus type 5 (Ad5), recombinant adenovirus (Ad) a replication-defective adenoviral vector system, is widely used for gene delivery in most cell types, and Genemedi got a rich experience in adenovirus packaging, you could find more information on https://www.genemedi.net/i/adenovirus-packaging .AAV-based gene transduction in vitro
As the most excellent gene therapy vector, recombinant AAV can mediate long-term stable expression of target genes in vitro/vivo with broad range of host and low immunity. For instance, AAV-DJ/8 was engineered via DNA family shuffling technology, displaying better in vitro transduction efficiencies compared to that of other wild type serotypes and higher infectivity rates in various cells and tissue types [13]; and AAV6 shows great transduction efficiency in CD4+ and CD8+ T cells [14]. Genemedi is good at AAV production, you can find more information and protocols about AAV on this website: www.genemedi.net/i/aav-packaging .Transfection in vivo
Transfection in vitro can be mediated by the non-viral reagents (such as liposome, polymer, nano-particle) or viral vectors (such as lentiviruses, adenovirus and AAV).Non-viral methods
Formulations of liposome, polymer and nano-particle have been optimized for in vivo delivery, especially with the use of transfection enhancer and secondary injection. What’s more, the three non-viral methods can also be combined together (Figure 1). As shown in Figure 1, multifunctional envelope-type nano-devices (MEND) are designed to promote the delivery of DNA and RNA. In MEND, PEG is used to extend nucleic acids’ half-life in systemic circulation, ligands are for specific targeting, peptides, including a protein-transduction domain, are intend to increase intracellular availability, while membrane fusogenic lipids were utilized to enhance endosomal escape and protect the degradation of nucleic acids [10,15].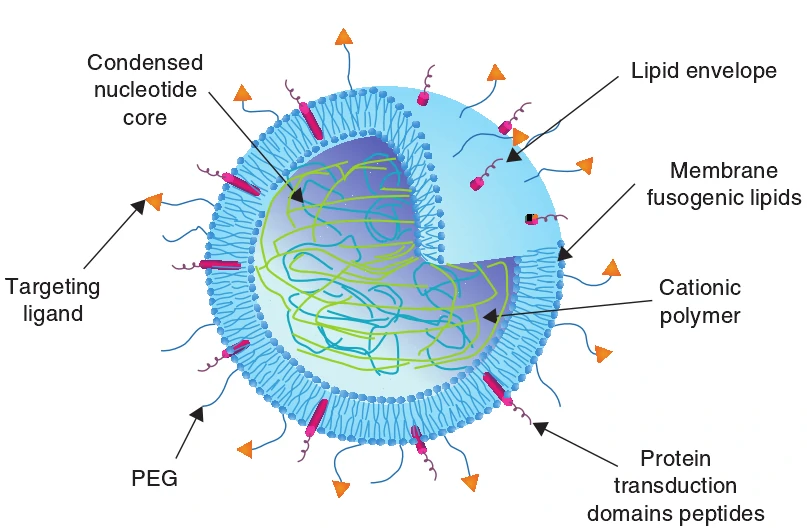
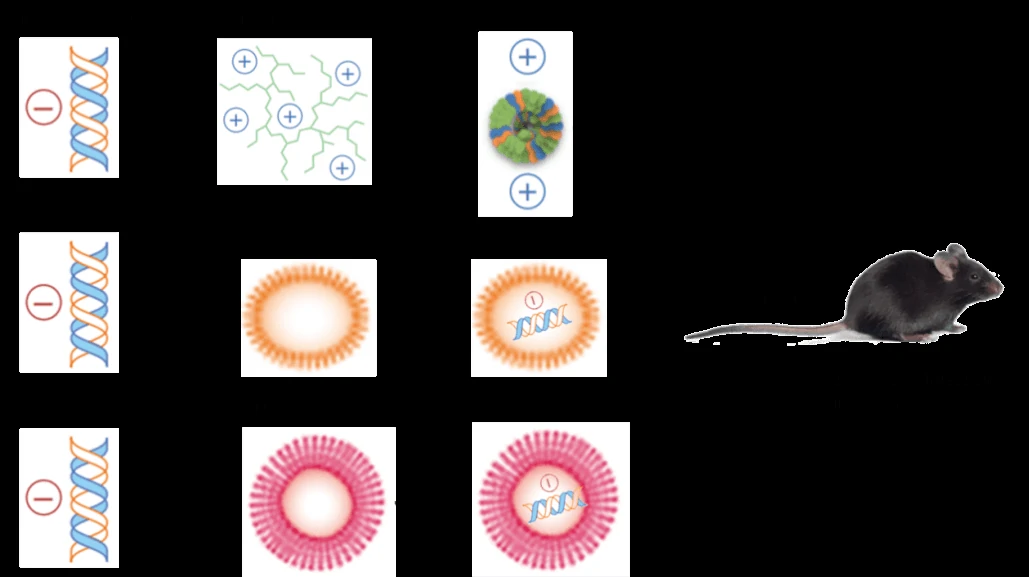
Viral vector
Lentiviruses can integrate a significant amount of viral cDNA into the host genome, mediate stable and long-term transgene expression, and efficiently infect dividing cells and nondividing cells in vivo [19]. You could find a lot of information and protocol about lentivirus on this website: https://www.genemedi.net/i/lentivirus-packaging.With no integration ability, recombinant adenovirus can’t be integrated into host genome, have large cargo capacity (~8kb), and are easily manipulated with recombinant DNA techniques [20]. Genemedi got a rich experience in adenovirus packaging and purification, you could find more information on https://www.genemedi.net/i/aav-packaging.
With mild immunogenicity, adeno-associated viruses (AAV) have superior biosafety rating and broad range of infectivity and mediate long-term and stable expression of target gene in vivo [21]. Genemedi is good at lentivirus production, please find more information on this website: https://www.genemedi.net/i/aav-packaging.
Comparison | Retrovirus | Lentivirus | Adenovirus | AAV |
Genome | ss RNA | ss RNA | ds DNA | ss DNA |
Integration | Yes | Yes | No | No |
Packaging Capacity | 3kb | 4kb | 5.5kb | 2kb |
Time to peak expression | 72h | 72h | 36h-72h | Cell: 7 days; Animals: 2 weeks |
Sustainable time | About 3 weeks | Stable expression | Transient expression | > 6 months |
Cell Type | Most Dividing | Most Dividing/Non-Dividing Cells | Most Dividing/Non-Dividing Cells | Most Dividing/Non-Dividing Cells |
Titer | 10^7 TU/ml | 10^8 TU/ml | 10^11 PFU/ml | 10^12 vg/ml |
Animal experiment | Suitable | Low efficiency | Lowest efficiency | Most suitable |
Immune Response | High | Medium | Medium | Ultra-low |
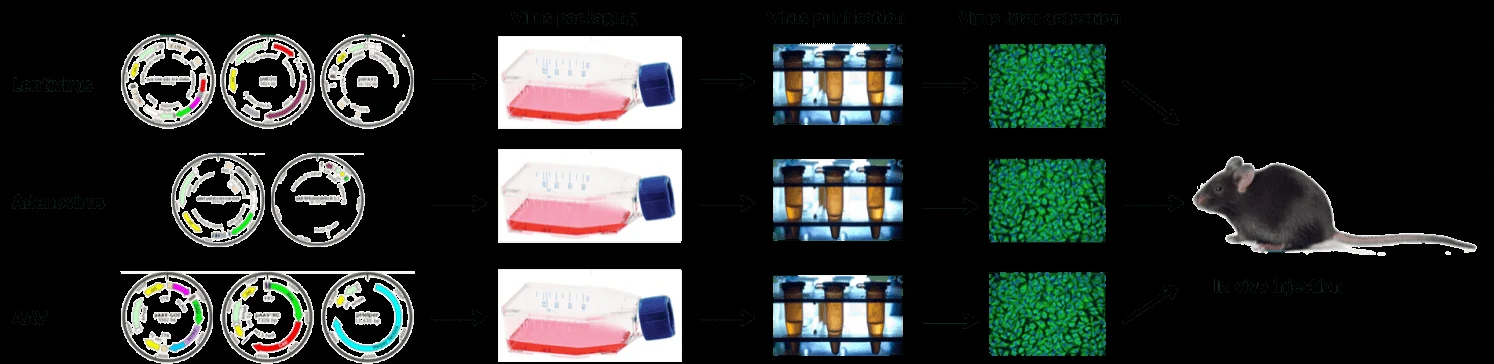
Small interfering RNA (siRNA) transfection
Small interfering RNA (siRNA) contains 21-25 nucleotides, specific for target RNA. Once entry into cells, siRNAs can be recruited into a multi-protein complex, known as RNA induced silencing complex (RISC), which interacts with the target RNA to mediate mRNA degradation, thus knock-down or suppress the expression of target gene [45]. siRNA transfection can be carried out with non-viral methods or viral vectors containing short hairpin RNA (shRNA), which can be subsequently processed into active siRNA.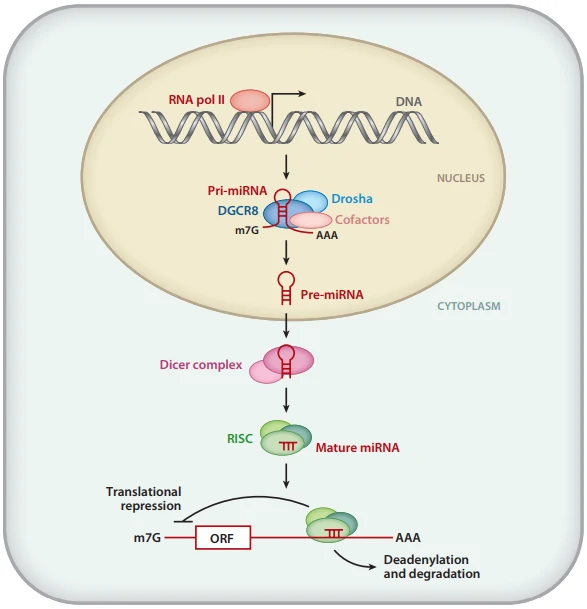
siRNA transfection in vitro
siRNA transient transfection in vitro can be mediated by polymer and liposome. Stable transfection cell lines need to be mediated by viral vectors (lentivirus, adenovirus, AAV) carry shRNA targeting gene of interest. The knock-down of shRNA varies in different sequence and needs to be tested. Besides, shRNA should be cloned into viral vectors for virus production. Therefore, viral-based stable transfection takes so much time but presents little cytotoxicity and high efficiency.siRNA transfection in vivo
Due to the instability of siRNA, low delivery efficiency and the superior difficulties in specific tissue distribution, in vivo siRNA transfection presents great technical challenges. Three methods have been adapted to deliver siRNA in vivo: 1) siRNAs with chemical modifications are generated, such as morpholino (MO) [47], 2'-deoxy-2'-fluoro-beta-D-arabinonucleic acid (FANA) [48], 2'-deoxy-2'-fluoro and 2'-O-methyl ribosugar modifications [49], Locked Nucleic Acid (LNA) modifications [50]; 2) encapsulation into liposomes also presents great protection from degradation in serum [51]; 3) viral vectors (lentivirus, adenovirus, AAV) containing shRNA targeting gene of interest show much higher delivery efficiency in vivo than chemical modifications or encapsulation, but these methods are time-consuming and might show some immunogenicity.Reference
1.
Liang X, J Potter, S Kumar, Y Zou, R Quintanilla, M Sridharan, J Carte, W Chen, N Roark, S Ranganathan, N Ravinder and JD Chesnut. (2015). Rapid and highly efficient mammalian cell engineering via Cas9 protein transfection. J Biotechnol 208:44-53.
2.
Suresh B, S Ramakrishna and H Kim. (2017). Cell-Penetrating Peptide-Mediated Delivery of Cas9 Protein and Guide RNA for Genome Editing. Methods Mol Biol 1507:81-94.
3.
Wirth T, N Parker and S Yla-Herttuala. (2013). History of gene therapy. Gene 525:162-9.
4.
Okita K, M Nakagawa, H Hyenjong, T Ichisaka and S Yamanaka. (2008). Generation of mouse induced pluripotent stem cells without viral vectors. Science 322:949-53.
5.
Kamimura K, T Suda, G Zhang and D Liu. (2011). Advances in Gene Delivery Systems. Pharmaceut Med 25:293-306.
6.
Fischer D, A von Harpe, K Kunath, H Petersen, Y Li and T Kissel. (2002). Copolymers of ethylene imine and N-(2-hydroxyethyl)-ethylene imine as tools to study effects of polymer structure on physicochemical and biological properties of DNA complexes. Bioconjug Chem 13:1124-33.
7.
Felgner PL, TR Gadek, M Holm, R Roman, HW Chan, M Wenz, JP Northrop, GM Ringold and M Danielsen. (1987). Lipofection: a highly efficient, lipid-mediated DNA-transfection procedure. Proc Natl Acad Sci U S A 84:7413-7.
8.
Felgner JH, R Kumar, CN Sridhar, CJ Wheeler, YJ Tsai, R Border, P Ramsey, M Martin and PL Felgner. (1994). Enhanced gene delivery and mechanism studies with a novel series of cationic lipid formulations. J Biol Chem 269:2550-61.
9.
Pipes BL, FH Vasanwala, TC Tsang, T Zhang, P Luo and DT Harris. (2005). Brief heat shock increases stable integration of lipid-mediated DNA transfections. Biotechniques 38:48, 50, 52.
10.
Akita H and H Harashima. (2008). Advances in non-viral gene delivery: using multifunctional envelope-type nano-device. Expert Opin Drug Deliv 5:847-59.
11.
Mann DG, TE McKnight, JT McPherson, PR Hoyt, AV Melechko, ML Simpson and GS Sayler. (2008). Inducible RNA interference-mediated gene silencing using nanostructured gene delivery arrays. ACS Nano 2:69-76.
12.
Bai HJ, P Zhang, L Ma, H Liang, G Wei and HT Yang. (2019). SMYD2 Drives Mesendodermal Differentiation of Human Embryonic Stem Cells Through Mediating the Transcriptional Activation of Key Mesendodermal Genes. Stem Cells.
13.
Hashimoto H, T Mizushima, T Chijiwa, M Nakamura and H Suemizu. (2017). Efficient production of recombinant adeno-associated viral vector, serotype DJ/8, carrying the GFP gene. Virus Res 238:63-68.
14.
Bak RO, DP Dever, A Reinisch, D Cruz Hernandez, R Majeti and MH Porteus. (2017). Multiplexed genetic engineering of human hematopoietic stem and progenitor cells using CRISPR/Cas9 and AAV6. Elife 6.
15.
Zylberberg C, K Gaskill, S Pasley and S Matosevic. (2017). Engineering liposomal nanoparticles for targeted gene therapy. Gene Ther 24:441-452.
16.
Aied A, U Greiser, A Pandit and W Wang. (2013). Polymer gene delivery: overcoming the obstacles. Drug Discov Today 18:1090-8.
17.
Montier T, T Benvegnu, PA Jaffres, JJ Yaouanc and P Lehn. (2008). Progress in cationic lipid-mediated gene transfection: a series of bio-inspired lipids as an example. Curr Gene Ther 8:296-312.
18.
Dobson J. (2006). Magnetic micro- and nano-particle-based targeting for drug and gene delivery. Nanomedicine (Lond) 1:31-7.
19.
Wu J, C Zheng, X Wang, S Yun, Y Zhao, L Liu, Y Lu, Y Ye, X Zhu, C Zhang, S Shi and Z Liu. (2015). MicroRNA-30 family members regulate calcium/calcineurin signaling in podocytes. J Clin Invest 125:4091-106.
20.
Liu TY, XQ Xiong, XS Ren, MX Zhao, CX Shi, JJ Wang, YB Zhou, F Zhang, Y Han, XY Gao, Q Chen, YH Li, YM Kang and GQ Zhu. (2016). FNDC5 Alleviates Hepatosteatosis by Restoring AMPK/mTOR-Mediated Autophagy, Fatty Acid Oxidation, and Lipogenesis in Mice. Diabetes 65:3262-3275.
21.
Li C, W Sun, C Gu, Z Yang, N Quan, J Yang, Z Shi, L Yu and H Ma. (2018). Targeting ALDH2 for Therapeutic Interventions in Chronic Pain-Related Myocardial Ischemic Susceptibility. Theranostics 8:1027-1041.
22.
Milone MC and U O'Doherty. (2018). Clinical use of lentiviral vectors. Leukemia 32:1529-1541.
23.
Cavazzana-Calvo M, E Payen, O Negre, G Wang, K Hehir, F Fusil, J Down, M Denaro, T Brady, K Westerman, R Cavallesco, B Gillet-Legrand, L Caccavelli, R Sgarra, L Maouche-Chretien, F Bernaudin, R Girot, R Dorazio, GJ Mulder, A Polack, A Bank, J Soulier, J Larghero, N Kabbara, B Dalle, B Gourmel, G Socie, S Chretien, N Cartier, P Aubourg, A Fischer, K Cornetta, F Galacteros, Y Beuzard, E Gluckman, F Bushman, S Hacein-Bey-Abina and P Leboulch. (2010). Transfusion independence and HMGA2 activation after gene therapy of human beta-thalassaemia. Nature 467:318-22.
24.
Cartier N, S Hacein-Bey-Abina, CC Bartholomae, G Veres, M Schmidt, I Kutschera, M Vidaud, U Abel, L Dal-Cortivo, L Caccavelli, N Mahlaoui, V Kiermer, D Mittelstaedt, C Bellesme, N Lahlou, F Lefrere, S Blanche, M Audit, E Payen, P Leboulch, B l'Homme, P Bougneres, C Von Kalle, A Fischer, M Cavazzana-Calvo and P Aubourg. (2009). Hematopoietic stem cell gene therapy with a lentiviral vector in X-linked adrenoleukodystrophy. Science 326:818-23.
25.
Biffi A, E Montini, L Lorioli, M Cesani, F Fumagalli, T Plati, C Baldoli, S Martino, A Calabria, S Canale, F Benedicenti, G Vallanti, L Biasco, S Leo, N Kabbara, G Zanetti, WB Rizzo, NA Mehta, MP Cicalese, M Casiraghi, JJ Boelens, U Del Carro, DJ Dow, M Schmidt, A Assanelli, V Neduva, C Di Serio, E Stupka, J Gardner, C von Kalle, C Bordignon, F Ciceri, A Rovelli, MG Roncarolo, A Aiuti, M Sessa and L Naldini. (2013). Lentiviral hematopoietic stem cell gene therapy benefits metachromatic leukodystrophy. Science 341:1233158.
26.
Sessa M, L Lorioli, F Fumagalli, S Acquati, D Redaelli, C Baldoli, S Canale, ID Lopez, F Morena, A Calabria, R Fiori, P Silvani, PM Rancoita, M Gabaldo, F Benedicenti, G Antonioli, A Assanelli, MP Cicalese, U Del Carro, MG Sora, S Martino, A Quattrini, E Montini, C Di Serio, F Ciceri, MG Roncarolo, A Aiuti, L Naldini and A Biffi. (2016). Lentiviral haemopoietic stem-cell gene therapy in early-onset metachromatic leukodystrophy: an ad-hoc analysis of a non-randomised, open-label, phase 1/2 trial. Lancet 388:476-87.
27.
Aiuti A, L Biasco, S Scaramuzza, F Ferrua, MP Cicalese, C Baricordi, F Dionisio, A Calabria, S Giannelli, MC Castiello, M Bosticardo, C Evangelio, A Assanelli, M Casiraghi, S Di Nunzio, L Callegaro, C Benati, P Rizzardi, D Pellin, C Di Serio, M Schmidt, C Von Kalle, J Gardner, N Mehta, V Neduva, DJ Dow, A Galy, R Miniero, A Finocchi, A Metin, PP Banerjee, JS Orange, S Galimberti, MG Valsecchi, A Biffi, E Montini, A Villa, F Ciceri, MG Roncarolo and L Naldini. (2013). Lentiviral hematopoietic stem cell gene therapy in patients with Wiskott-Aldrich syndrome. Science 341:1233151.
28.
Sweeney K and G Hallden. (2016). Oncolytic adenovirus-mediated therapy for prostate cancer. Oncolytic Virother 5:45-57.
29.
Castro JE, J Melo-Cardenas, M Urquiza, JS Barajas-Gamboa, RS Pakbaz and TJ Kipps. (2012). Gene immunotherapy of chronic lymphocytic leukemia: a phase I study of intranodally injected adenovirus expressing a chimeric CD154 molecule. Cancer Res 72:2937-48.
30.
Predina JD, J Keating, O Venegas, S Nims and S Singhal. (2016). Neoadjuvant intratumoral immuno-gene therapy for non-small cell lung cancer. Discov Med 21:275-81.
31.
Schiza A, J Wenthe, S Mangsbo, E Eriksson, A Nilsson, TH Totterman, A Loskog and G Ullenhag. (2017). Adenovirus-mediated CD40L gene transfer increases Teffector/Tregulatory cell ratio and upregulates death receptors in metastatic melanoma patients. J Transl Med 15:79.
32.
Garcia-Carbonero R, R Salazar, I Duran, I Osman-Garcia, L Paz-Ares, JM Bozada, V Boni, C Blanc, L Seymour, J Beadle, S Alvis, B Champion, E Calvo and K Fisher. (2017). Phase 1 study of intravenous administration of the chimeric adenovirus enadenotucirev in patients undergoing primary tumor resection. J Immunother Cancer 5:71.
33.
Kassner U, T Hollstein, T Grenkowitz, M Wuhle-Demuth, B Salewsky, I Demuth, M Dippel and E Steinhagen-Thiessen. (2018). Gene Therapy in Lipoprotein Lipase Deficiency: Case Report on the First Patient Treated with Alipogene Tiparvovec Under Daily Practice Conditions. Hum Gene Ther 29:520-527.
34.
Passini MA, J Bu, AM Richards, CM Treleaven, JA Sullivan, CR O'Riordan, A Scaria, AP Kells, L Samaranch, W San Sebastian, T Federici, MS Fiandaca, NM Boulis, KS Bankiewicz, LS Shihabuddin and SH Cheng. (2014). Translational fidelity of intrathecal delivery of self-complementary AAV9-survival motor neuron 1 for spinal muscular atrophy. Hum Gene Ther 25:619-30.
35.
Stieger K and B Lorenz. (2014). [Specific gene therapy for hereditary retinal dystrophies - an update]. Klin Monbl Augenheilkd 231:210-5.
36.
Trapani I, P Colella, A Sommella, C Iodice, G Cesi, S de Simone, E Marrocco, S Rossi, M Giunti, A Palfi, GJ Farrar, R Polishchuk and A Auricchio. (2014). Effective delivery of large genes to the retina by dual AAV vectors. EMBO Mol Med 6:194-211.
37.
Doi K and Y Takeuchi. (2015). Gene therapy using retrovirus vectors: vector development and biosafety at clinical trials. Uirusu 65:27-36.
38.
Duncan GA, N Kim, Y Colon-Cortes, J Rodriguez, M Mazur, SE Birket, SM Rowe, NE West, A Livraghi-Butrico, RC Boucher, J Hanes, G Aslanidi and JS Suk. (2018). An Adeno-Associated Viral Vector Capable of Penetrating the Mucus Barrier to Inhaled Gene Therapy. Mol Ther Methods Clin Dev 9:296-304.
39.
Bowles DE, SW McPhee, C Li, SJ Gray, JJ Samulski, AS Camp, J Li, B Wang, PE Monahan, JE Rabinowitz, JC Grieger, L Govindasamy, M Agbandje-McKenna, X Xiao and RJ Samulski. (2012). Phase 1 gene therapy for Duchenne muscular dystrophy using a translational optimized AAV vector. Mol Ther 20:443-55.
40.
Nathwani AC, EG Tuddenham, S Rangarajan, C Rosales, J McIntosh, DC Linch, P Chowdary, A Riddell, AJ Pie, C Harrington, J O'Beirne, K Smith, J Pasi, B Glader, P Rustagi, CY Ng, MA Kay, J Zhou, Y Spence, CL Morton, J Allay, J Coleman, S Sleep, JM Cunningham, D Srivastava, E Basner-Tschakarjan, F Mingozzi, KA High, JT Gray, UM Reiss, AW Nienhuis and AM Davidoff. (2011). Adenovirus-associated virus vector-mediated gene transfer in hemophilia B. N Engl J Med 365:2357-65.
41.
Jessup M, B Greenberg, D Mancini, T Cappola, DF Pauly, B Jaski, A Yaroshinsky, KM Zsebo, H Dittrich, RJ Hajjar and I Calcium Upregulation by Percutaneous Administration of Gene Therapy in Cardiac Disease. (2011). Calcium Upregulation by Percutaneous Administration of Gene Therapy in Cardiac Disease (CUPID): a phase 2 trial of intracoronary gene therapy of sarcoplasmic reticulum Ca2+-ATPase in patients with advanced heart failure. Circulation 124:304-13.
42.
LeWitt PA, AR Rezai, MA Leehey, SG Ojemann, AW Flaherty, EN Eskandar, SK Kostyk, K Thomas, A Sarkar, MS Siddiqui, SB Tatter, JM Schwalb, KL Poston, JM Henderson, RM Kurlan, IH Richard, L Van Meter, CV Sapan, MJ During, MG Kaplitt and A Feigin. (2011). AAV2-GAD gene therapy for advanced Parkinson's disease: a double-blind, sham-surgery controlled, randomised trial. Lancet Neurol 10:309-19.
43.
Aalbers CJ, L Bevaart, S Loiler, K de Cortie, JF Wright, F Mingozzi, PP Tak and MJ Vervoordeldonk. (2015). Preclinical Potency and Biodistribution Studies of an AAV 5 Vector Expressing Human Interferon-beta (ART-I02) for Local Treatment of Patients with Rheumatoid Arthritis. PLoS One 10:e0130612.
44.
Bevaart L, CJ Aalbers, MP Vierboom, N Broekstra, I Kondova, E Breedveld, B Hauck, JF Wright, PP Tak and MJ Vervoordeldonk. (2015). Safety, Biodistribution, and Efficacy of an AAV-5 Vector Encoding Human Interferon-Beta (ART-I02) Delivered via Intra-Articular Injection in Rhesus Monkeys with Collagen-Induced Arthritis. Hum Gene Ther Clin Dev 26:103-12.
45.
Mawji IA and PA Marsden. (2006). RNA transfection is a versatile tool to investigate endothelin-1 posttranscriptional regulation. Exp Biol Med (Maywood) 231:704-8.
46.
Hata A. (2013). Functions of microRNAs in cardiovascular biology and disease. Annu Rev Physiol 75:69-93.
47.
Negri A, M Ferrari, R Nodari, E Coppa, V Mastrantonio, S Zanzani, D Porretta, C Bandi, S Urbanelli and S Epis. (2019). Gene silencing through RNAi and antisense Vivo-Morpholino increases the efficacy of pyrethroids on larvae of Anopheles stephensi. Malar J 18:294.
48.
Dowler T, D Bergeron, AL Tedeschi, L Paquet, N Ferrari and MJ Damha. (2006). Improvements in siRNA properties mediated by 2'-deoxy-2'-fluoro-beta-D-arabinonucleic acid (FANA). Nucleic Acids Res 34:1669-75.
49.
Foster DJ, CR Brown, S Shaikh, C Trapp, MK Schlegel, K Qian, A Sehgal, KG Rajeev, V Jadhav, M Manoharan, S Kuchimanchi, MA Maier and S Milstein. (2018). Advanced siRNA Designs Further Improve In Vivo Performance of GalNAc-siRNA Conjugates. Mol Ther 26:708-717.
50.
Subramanian N, JR Kanwar, RK Kanwar and S Krishnakumar. (2015). Targeting Cancer Cells Using LNA-Modified Aptamer-siRNA Chimeras. Nucleic Acid Ther 25:317-22.
51.
Koide H, A Okamoto, H Tsuchida, H Ando, S Ariizumi, C Kiyokawa, M Hashimoto, T Asai, T Dewa and N Oku. (2016). One-step encapsulation of siRNA between lipid-layers of multi-layer polycation liposomes by lipoplex freeze-thawing. J Control Release 228:1-8.